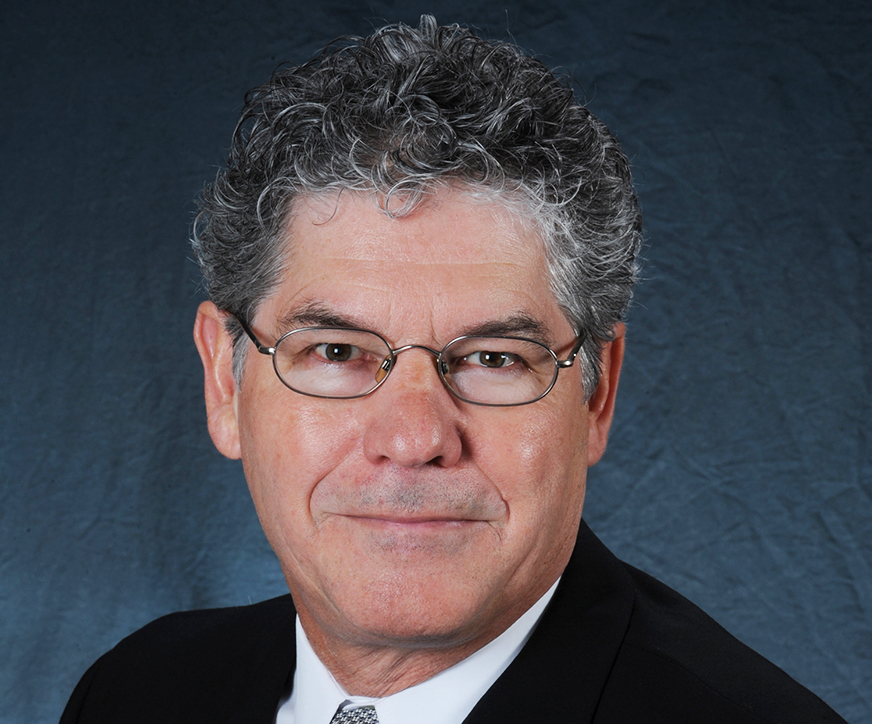
J. Michael Ramsey
Minnie N. Goldby Distinguished Professor of Chemistry
Chapman Hall 251
jmramsey@unc.edu
919-965-7492
Research Interest:
Microfabricated Chemical Instrumentation, Microfluidics, Nanofluidics
Professional Background
B.S. Chemistry, Bowling Green State University, 1974; Ph.D. Chemistry, Indiana University, 1979; Eugene P. Wigner Distinguished Postdoctoral Fellow, Oak Ridge National Laboratory, 1979; Research Staff, Oak Ridge National Laboratory, 1981; Group Leader, Oak Ridge National Laboratory, 1985; Alumnus of the Year, Department of Chemistry, Bowling Green State University, 1992; Founding Scientist, Caliper Technologies, Inc., 1995; Scientist of the Year Award, Oak Ridge National Laboratory, 1996; Discover Magazine Annual Technology Award, 1996; R&D 100 Award, Lab-on-a-Chip Technology, 1996; Corporate Research Fellow, Oak Ridge National Laboratory, 1997; Fellow, Optical Society of America, 1998; Alexander von Humboldt Award for Senior Scientist, 1999; Frederick Conference Capillary Electrophoresis Award, 2000; Desty Memorial Lecture, The Royal Institution, 2000; R&D 100 Top 40, Lab-on-a-Chip Technology, 2001; Energy@23 Award, 2001; A. J. P. Martin Gold Medal for Separation Science, 2001; Jacob Heskel Gabbay Award in Biotechnology and Medicine, 2001; Battelle Distinguished Inventor Award, 2003; Marcel J. E. Golay Award in Capillary Chromatography, 2003; ACS Division of Analytical Chemistry Award in Chemical Instrumentation, 2003; R&D 100 Award, μTrapMS, 2003; Federal Laboratory Consortium Excellence in Technology Transfer Award, 2004; Pittsburgh Analytical Chemistry Award, 2006; American Chemical Society Award in Chromatography, 2007; Fellow, American Institute for Medical and Biological Engineering, 2008, Fellow, American Chemical Society, 2011; The CASSS Award for Outstanding Achievements in Separation Science, 2012; Ralph N. Adams Award in Bioanalytical Chemistry, 2013; Member, National Academy of Engineering, 2014
Research Synopsis
We are interested in utilizing micro- and nanofabrication strategies to create devices that facilitate our abilities to gather chemical and biochemical information. Our motivations for fabricating devices include point of care clinical diagnostics, high-throughput biochemical experimentation, development of new types of chemical sensors, and understanding of transport mechanisms in nanoscale-confined spaces. The devices that we develop have application to drug discovery, health care, environmental monitoring, and basic research.
Many of our projects involve the microfabrication of fluidic networks that are used to perform biochemical assays. This area of research is often referred to as microfluidics, microchips, or Lab-on-a-Chip technology. We are presently interested in developing microfluidic devices for addressing clinical diagnostics, proteomics problems, and automated single cell assays. Proteomics is a term used to describe the understanding of the full complement of proteins expressed by an organism. A given type of cell will contain several thousand proteins and the task of identifying all proteins is a significant challenge. Traditional approaches to solving this problem involve the use of milligram quantities of protein and chemical separations that unfold over a period of days. We are pursuing microfabricated fluidics approaches to implementing multidimensional liquid phase separations that utilize nanogram to picogram quantities of materials with separations unfolding over a period of tens of minutes. The ability to perform electrospray ionization from microchips is also being developed to allow structural analysis via mass spectrometry of the isolated proteins.
Microfluidics devices are also being developed to autonomously manipulate single non-adherent cells, characterize them using flow cytometry techniques, lyse the cells and chemical analyze the cell contents utilizing chemical separations techniques. This automated approach allows high-throughput characterization at the single cell level so that statistics can be rapidly generated on cell heterogeneity. Through collaboration with Prof. Nancy Allbritton, UNC-CH, multiple kinase assays are being implemented on these devices to study cell signal transduction mechanisms.
More fundamental in nature is the study of molecular transport through conduits with nanometer scale dimensions, what we refer to as nanofluidics. We are studying both fluid and polymer transport through individual nanoscale conduits or pores that are top-down fabricated in hard materials such as glass, quartz, or silicon. We are attempting to further reduce lateral dimensions of channels and pores utilizing bottom-up strategies and interfacing molecular assemblies to features formed in hard materials. While this work is fundamental, understanding could lead to new methods for the separation and analysis of polymeric materials, new types of chemical sensors, or possibly technology that allows the structure of single polymer molecules to be determined, e.g., single molecule DNA sequencing.
We are also investigating the prospects of shrinking the size of conventional mass spectrometry by several orders of magnitude. Specifically we have been experimentally exploring the reduction in size of Paul-type ion trap mass analyzers and assessing their performance. Reduction in length scale of this type of mass spectrometer is particularly interesting because the mass resolution of the device does not fundamentally depend on its size. Microscale ion trap MS devices that are a factor of 40 smaller than conventional scale have been operated with no loss in mass resolving power. Microfabricated ion trap devices with fundamental length scales as small as 10 μm have been demonstrated. Smaller mass analyzers also allow operation at lower ion mean-free path, higher pressures. Mass spectra have been generated at pressures exceeding 1 torr, greatly reducing pumping requirements. Miniature mass spectrometers have application to problems such as environmental monitoring, chemical process control, and high-throughput laboratory analysis.